Measuring Principle Definition
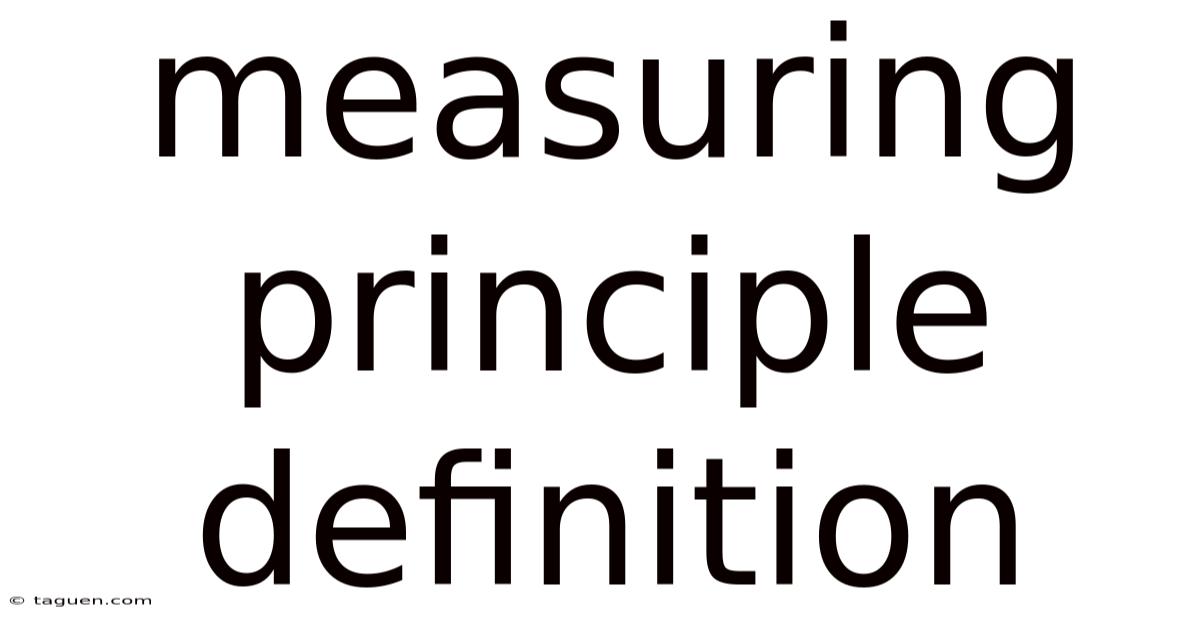
Discover more detailed and exciting information on our website. Click the link below to start your adventure: Visit Best Website meltwatermedia.ca. Don't miss out!
Table of Contents
Decoding the Essence: A Deep Dive into Measurement Principles
What if the accuracy of every scientific discovery, engineering marvel, and technological advancement hinges on a precise understanding of measurement principles? These foundational principles underpin our ability to quantify the world, driving innovation and shaping our understanding of reality.
Editor’s Note: This article on measurement principles has been updated today, providing the latest insights and expert analysis in the field.
Understanding measurement principles is fundamental to virtually every aspect of modern life. From the precise calibration of medical equipment to the accurate tracking of global climate change, the ability to measure accurately and reliably is paramount. This article explores the core concepts, applications, challenges, and future implications of measurement principles, providing actionable knowledge for professionals and enthusiasts alike. This exploration will encompass various fields, highlighting the unifying principles that govern accurate and reliable measurements.
This article delves into the core aspects of measurement principles, examining their relevance, real-world applications, and future potential. Backed by expert insights and data-driven research, it provides actionable knowledge for industry professionals and enthusiasts alike. We will explore the definition and core concepts, applications across industries, challenges and solutions, and the impact on innovation.
The Pillars of Measurement: Definition and Core Concepts
Measurement, at its core, is the process of assigning a numerical value to a physical quantity or attribute. This seemingly simple definition encompasses a complex interplay of theoretical frameworks, practical techniques, and technological advancements. Key core concepts underpinning this process include:
- Measurand: The physical quantity or attribute being measured (e.g., length, mass, temperature, voltage).
- Units: Standardized quantities used for comparison and quantification (e.g., meters, kilograms, Kelvin, volts). The International System of Units (SI) provides a globally accepted framework for these units.
- Measurement Standard: A physical object or phenomenon with precisely known properties, used to calibrate measuring instruments. These standards are crucial for ensuring consistency and accuracy across different measurements.
- Measurement Uncertainty: An expression of the doubt associated with a measurement result. It quantifies the range within which the true value is likely to lie. Understanding and minimizing uncertainty is a critical aspect of reliable measurement.
- Calibration: The process of comparing a measuring instrument's readings to a known standard to ensure accuracy. Regular calibration is essential for maintaining the reliability of measurement systems.
- Traceability: The ability to link a measurement result back to a national or international standard, ensuring consistency and comparability across different locations and times.
Applications Across Industries: A Spectrum of Precision
The applications of precise measurement are ubiquitous and diverse. Consider the following examples:
- Healthcare: Accurate measurement of vital signs (blood pressure, heart rate, temperature), blood glucose levels, and drug dosages is crucial for diagnosis, treatment, and patient monitoring. Medical imaging techniques, such as MRI and CT scans, rely on precise measurements for creating detailed images of the human body.
- Manufacturing: Precision manufacturing relies heavily on accurate measurements to ensure quality control, optimize production processes, and meet stringent specifications. This applies across various industries, from automotive and aerospace to electronics and pharmaceuticals. Dimensional metrology, for instance, plays a critical role in ensuring component tolerances.
- Environmental Monitoring: Accurate measurements of air and water quality, greenhouse gas concentrations, and other environmental parameters are vital for understanding and mitigating environmental challenges. Remote sensing technologies and in-situ measurements are essential for monitoring these variables.
- Scientific Research: Scientific research relies heavily on accurate measurements to validate hypotheses, test theories, and develop new knowledge. Measurements in fields such as physics, chemistry, and biology often require highly specialized instruments and techniques.
- Finance: Accurate measurement of financial indicators, such as stock prices, interest rates, and currency exchange rates, is essential for investment decisions, risk management, and economic modeling.
Challenges and Solutions: Navigating the Complexities of Measurement
Despite the advancements in measurement technologies, several challenges remain:
- Uncertainty and Error: Minimizing measurement uncertainty is an ongoing challenge. Sources of error can include systematic errors (biases) and random errors (fluctuations). Statistical methods are used to analyze and reduce these errors.
- Calibration and Traceability: Maintaining the traceability of measurements to national or international standards is crucial for ensuring consistency and comparability. This requires robust calibration procedures and traceability chains.
- Technological Limitations: Certain measurements, such as those at extremely small or large scales, require specialized technologies that may have limitations in terms of accuracy or precision.
- Environmental Factors: Environmental factors such as temperature, pressure, and humidity can affect the accuracy of measurements. Compensation techniques are needed to mitigate these effects.
- Human Error: Human error, such as incorrect instrument reading or data entry, can significantly impact measurement accuracy. Careful training and standardized procedures are essential to minimize human error.
The Impact on Innovation: A Driving Force of Progress
The pursuit of increasingly accurate and precise measurements has been a driving force of innovation across many fields. Advancements in measurement technologies have led to breakthroughs in various areas:
- Nanotechnology: The ability to measure at the nanoscale has enabled the development of novel materials and devices with unique properties. Techniques such as atomic force microscopy and scanning tunneling microscopy are essential for characterizing nanomaterials.
- Biotechnology: Advancements in biosensors and other measurement technologies have revolutionized biotechnology, enabling the development of new diagnostic tools, therapies, and research methods.
- Information Technology: Precise measurements of electrical signals and data transmission rates are essential for the development of high-speed computer networks and other information technologies.
- Renewable Energy: Accurate measurement of solar radiation, wind speed, and other renewable energy resources is critical for optimizing the design and operation of renewable energy systems.
Key Takeaways: A Summary of Essential Insights
Key Aspect | Description |
---|---|
Measurand | The physical quantity or attribute being measured. |
Units & SI System | Standardized quantities for comparison; the internationally accepted system of units. |
Measurement Uncertainty | The doubt associated with a measurement result. |
Calibration & Traceability | Comparing to standards; linking results back to national or international standards. |
Applications | Healthcare, manufacturing, environmental monitoring, scientific research, finance, and many others. |
Challenges | Uncertainty, error, technological limitations, environmental factors, and human error. |
Impact on Innovation | Driving force behind advancements in nanotechnology, biotechnology, information technology, and more. |
With a strong understanding of its relevance, let’s explore measurement principles further, uncovering their applications, challenges, and future implications.
Exploring the Interplay Between Precision and Accuracy in Measurement Principles
Precision and accuracy are often confused, but they represent distinct aspects of measurement quality. Precision refers to the closeness of repeated measurements to each other, while accuracy refers to how close a measurement is to the true value. High precision doesn't necessarily imply high accuracy; a highly precise measurement could be consistently off by a significant amount if the instrument is not properly calibrated. The interplay between these two factors is crucial for ensuring reliable measurements.
Roles and Real-World Examples:
- Manufacturing: In manufacturing, high precision is crucial for producing parts with consistent dimensions. However, high accuracy is essential to ensure that these dimensions meet the specified tolerances. A high-precision machine tool that is not accurately calibrated will produce parts that are consistently off-target, leading to quality issues.
- Scientific Research: In scientific research, both precision and accuracy are essential for reliable results. Repeated measurements with high precision help to reduce random errors, while accurate measurements minimize systematic errors. For example, in experiments involving astronomical observations, high precision in measuring the positions of stars is necessary to detect subtle movements, while accuracy is crucial for ensuring that the measurements are not affected by systematic errors such as atmospheric refraction.
Risks and Mitigations:
- Low Precision: Low precision can lead to unreliable results, making it difficult to draw conclusions from experiments or to control manufacturing processes effectively. This can be mitigated by using more precise instruments, improving measurement techniques, and employing statistical methods to analyze the data.
- Low Accuracy: Low accuracy can lead to significant errors in measurements, leading to incorrect conclusions or faulty products. This can be addressed by calibrating instruments regularly, using appropriate measurement techniques, and minimizing systematic errors.
Impact and Implications:
The pursuit of both high precision and high accuracy is driving advancements in measurement technologies and techniques. New instruments and methods are continuously being developed to achieve higher levels of both precision and accuracy, leading to improved outcomes in various fields.
Conclusion: The Enduring Significance of Measurement Principles
Measurement principles form the bedrock of scientific discovery, technological advancement, and societal progress. The ability to accurately and reliably quantify the world around us is essential for addressing global challenges, fostering innovation, and improving the quality of life. By understanding the core concepts, applications, and challenges associated with measurement, we can strive towards even more precise and reliable measurements, driving further breakthroughs across diverse fields. The continuous pursuit of improved measurement techniques and technologies ensures our continued ability to understand and shape our world with increasing accuracy.
Further Analysis: A Deep Dive into Calibration Techniques
Calibration is a critical process that ensures the accuracy of measuring instruments. Several calibration techniques exist, each with its own strengths and limitations:
- Direct Comparison: This involves directly comparing the readings of a measuring instrument to a known standard. This is a simple and effective method for many instruments.
- Substitution: In substitution calibration, the unknown quantity is substituted with a known standard, and the instrument's response is compared. This minimizes the influence of the instrument's own characteristics.
- Comparison with a Secondary Standard: This involves calibrating an instrument against a secondary standard that has been previously calibrated against a primary standard. This is often more practical than direct comparison with a primary standard.
- In-Situ Calibration: This involves calibrating an instrument while it is in its operational environment. This accounts for environmental factors that might affect the measurement.
Frequently Asked Questions (FAQs): Addressing Common Queries
-
What is the difference between precision and accuracy? Precision refers to the repeatability of measurements, while accuracy refers to how close measurements are to the true value.
-
What are the different types of measurement errors? Measurement errors can be systematic (biases) or random (fluctuations).
-
How important is calibration? Calibration is crucial for maintaining the accuracy and reliability of measuring instruments.
-
What is traceability in measurement? Traceability ensures that a measurement can be linked back to a national or international standard.
-
How can measurement uncertainty be reduced? Measurement uncertainty can be reduced through careful experimental design, precise instrument selection, and statistical analysis.
-
What are some emerging trends in measurement technology? Emerging trends include nanometrology, biosensors, and advanced imaging techniques.
Practical Tips for Maximizing the Benefits of Measurement Principles:
- Use calibrated instruments: Ensure all measuring instruments are regularly calibrated and traceable to national or international standards.
- Follow proper measurement techniques: Adhere to established protocols and procedures to minimize measurement errors.
- Employ statistical methods: Use statistical techniques to analyze data, identify outliers, and reduce uncertainty.
- Account for environmental factors: Consider environmental conditions that may affect measurements and implement appropriate compensation techniques.
- Document all measurements: Maintain detailed records of measurements, including date, time, instrument used, and any relevant environmental conditions.
- Regularly review and update procedures: Stay abreast of advancements in measurement techniques and technologies to optimize measurement processes.
- Utilize appropriate data analysis techniques: Select the correct statistical methods for your data to properly assess and interpret the results.
- Invest in training: Ensure personnel are properly trained in measurement techniques and the use of measuring instruments.
Conclusion: Embracing the Power of Precise Measurement
With its transformative potential, the understanding and application of measurement principles are shaping the future across various industries. By embracing its principles and addressing challenges, businesses, researchers, and individuals can unlock new opportunities for growth, innovation, and a deeper understanding of the world. The pursuit of ever-increasing precision and accuracy will continue to fuel progress and discovery for generations to come.
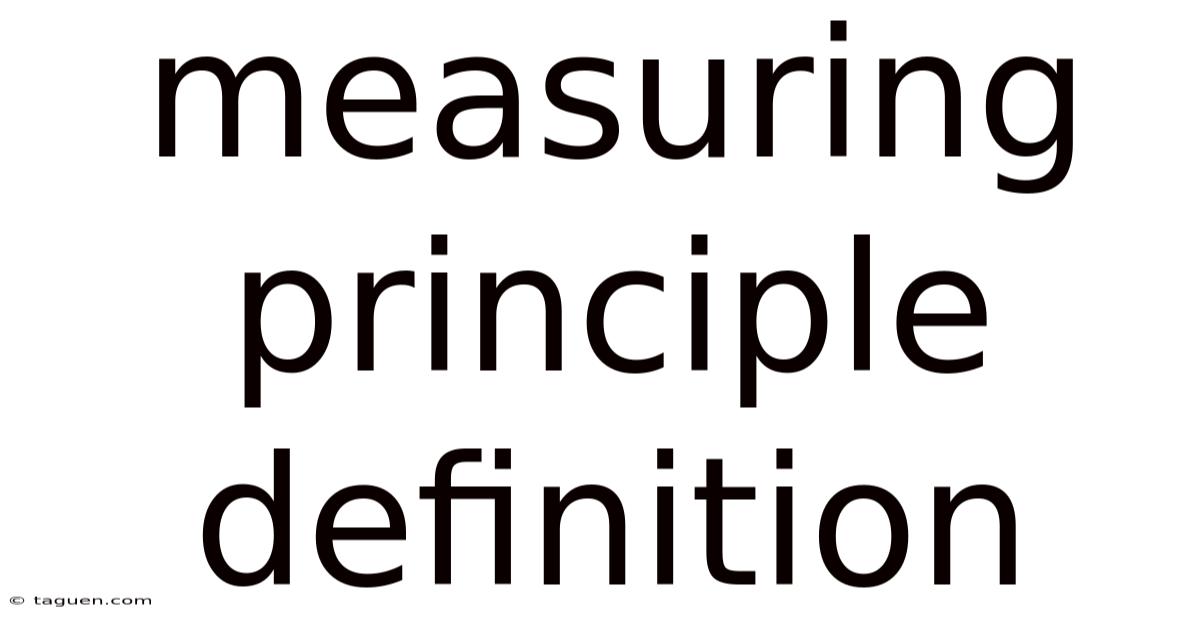
Thank you for visiting our website wich cover about Measuring Principle Definition. We hope the information provided has been useful to you. Feel free to contact us if you have any questions or need further assistance. See you next time and dont miss to bookmark.
Also read the following articles
Article Title | Date |
---|---|
Market Letter Definition | Apr 16, 2025 |
How Hard Is It To Learn Forex Trading | Apr 16, 2025 |
What Are Perpetual Futures Contracts | Apr 16, 2025 |
Market Approach Definition And How It Works To Value An Asset | Apr 16, 2025 |
Market Value Clause Definition | Apr 16, 2025 |